Mitigating Emissions and Adapting to Climate Change Through Regenerative Organic Agriculture
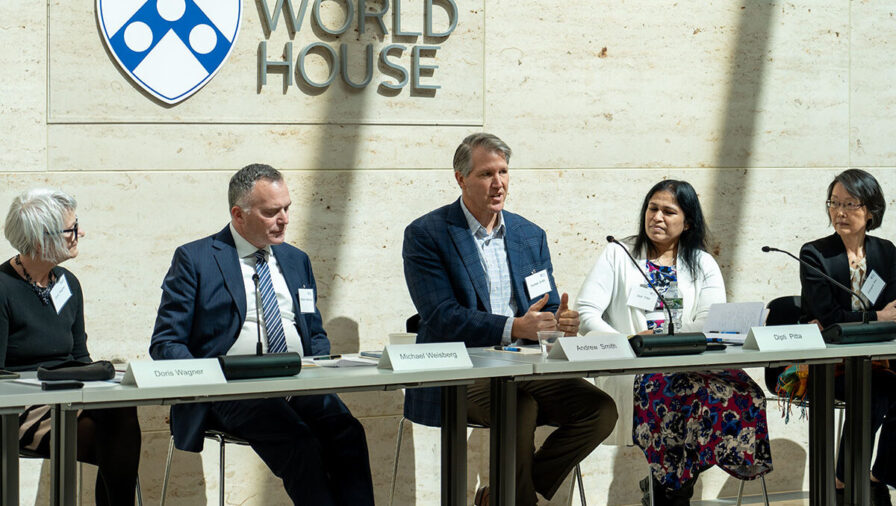
Background
Organic farming provides multiple sustainability benefits [1] but evidence for organic farming as a climate solution is mixed. It has been proposed that organic production systems provide a climate benefit through increased soil carbon sequestration [2] and reduced greenhouse gas emissions compared to conventional forms of agriculture [3].
Introduction
Long-term studies comparing organic and conventional management find higher soil carbon stocks in the organically managed soils with incremental soil carbon increases over time [2, 4-8] suggesting that conversion to organic agriculture could sequester carbon in the short-term until soil carbon levels reach equilibrium [9]. On an area-scaled basis (tonnes CO2-eq ha-1 yr-1), organic farming has lower greenhouse gas emissions (N2O, CH4, CO2) than conventional forms of farming [10, 11]. Considering these factors, organic agriculture appears to be a win-win climate mitigation solution for the agricultural and food sectors. However, these data are confounded by several factors and additional investigation is needed before confirming organic farming as a verifiable climate solution. First, it has been noted that higher soil carbon stocks is largely the result of increased applications of organic fertility sources such as manure and compost which is a diversion of carbon from waste-streams rather than atmospheric sequestration, and thus cannot be considered a climate mitigation strategy [12]. Furthermore, scaling of organic farming using livestock manure would require an increase of animal agriculture which is contrary to most proposed climate solutions [13]. Second, when greenhouse gas calculations are converted to a yield-scale basis (tonnes CO2-eq kgcrop-1), organic farming may lead to higher greenhouse gas emissions compared to conventional forms of farming due to lower yields in the organic systems [14, 15] although results are conflicting [16]. Diversified organic systems reduce the organic-conventional yield gap [15, 17] and therefore may result in an overall reduced greenhouse gas emissions on an area and yield scale basis, despite manure application within those systems [15; M. Graham, personal communication]. Also, emissions from manure application in organic and conventional systems is not equal [11]. Organic systems tend to be nitrogen limiting while conventional systems tend to be nitrogen rich. For example, application of manures in two organic system resulted in a 40.2 percent decline in emissions compared to conventional inorganic fertilizer application while the combined use of inorganic fertilizer and manure in a conventional cropping system led to higher methane emissions than inorganic fertility alone [11]. It has been proposed that overcoming this nitrogen limitation in organic farming, potentially with increased fertilizer application, would reduce the yield gap [18] and could lead to more favorable global greenhouse gas calculations for organic farming through increased crop yields. However, the question remains, how to achieve agricultural intensification in a sustainable manner without simultaneously increasing greenhouse gas emissions?
The Rodale Institute Farming Systems Trial
Rodale Institute has begun to explore the potential for fossil fuel free farming and sustainable crop intensification through regenerative organic agriculture. A recent carbon footprint analysis of the Farming Systems Trial (Kutztown, PA) revealed gaps in knowledge and future directions for this ambitious goal. The Farming Systems Trial is the longest running side-by-side comparison of organic and conventional grain cropping systems in North America. Started in 1981, the experiment includes a conventional (CONV) corn-soybean rotation that represents the majority of the US agricultural land base, and two organic systems – a low-input, legume based system (LEG) that relies solely on leguminous green manures as sources of fertility, and a manure based system (MNR) that relies on green and livestock manures as a source of fertility. The organic systems are also more diverse with the LEG system following a four-year crop rotation and the MNR system a nine-year rotation. The improvements of soil health and increased soil carbon in the organically managed soils has been well documented over the years [5, 7, 19, 20]. Other documented benefits of organic management include complete elimination of herbicides, insecticides, and fungicides, reduced nitrate leaching [4], reduced energy use [21], higher profits [17, 21], and similar crop yields to conventional management [17, 21, 22], especially during periods of drought [23]. The latter finding of higher higher yields during periods of low rainfall suggests organic farming will be more resilient to climate fluctuations. Similar yields between the organic and conventional systems have resulted in a reduced carbon footprint from the organic systems on an area-scaled and yield-scaled basis.
Carbon Footprint of Conventional and Diversified Organic Farming Systems
A carbon footprint analysis was performed on all farming systems to estimate greenhouse gas emissions and identify gaps in analysis and areas for sustainable intensification (M. Graham, personal communication). Absolute, area-scaled emissions were highest for the conventional system (1.25 – 1.72 tonnes CO2-eq ha-1 yr-1), whereas emissions were 25 to 37 percent lower in the organic MNR system (0.94 – 1.09 tonnes CO2-eq ha-1 yr-1) and 52 to 74 percent lower in the organic LEG system (0.33 – 0.83 tonnes CO2-eq ha-1 yr-1). Yield-scaled emissions of maize in the baseline analyses were highest in the conventional system (0.19 – 0.26 kg CO2-eq kg-1), followed by the organic manure (0.13 – 0.16 kg CO2-eq kg-1) and organic legume (0.07 – 0.17 kg CO2-eq kg-1). Despite lower yields in the LEG system, yield-scaled emissions on a feed digestible energy basis were highest in CNV (0.014 – 0.020 kg CO2-eq MJ-1) but were similar between MNR (0.009 – 0.010 kg CO2-eq MJ-1) and LEG (0.006 – 0.015 kg CO2-eq MJ-1). The highest emissions factors for the CNV system included N2O from application of nitrogen fertilizer and the manufacturing of fertilizers and pesticides. The highest emission factor for the MNR system resulted from composted manure application. This has been identified as a major research gap as few studies have measured greenhouse gases from organic fertility sources in the field [11, 24] and current models may be overinflating emissions from these fertility sources. While refining models may make organic more favorable as a climate solution, it still does not achieve zero emissions or energy independence from fossil fuels. While the benefits of compost as a soil amendment that improves soil and plant health has been well documented, this analysis identifies that alternative forms of organic fertility are needed to scale organic farming in a sustainable and climate-friendly manner.
Alternative Fertility Sources to Achieve Fossil Fuel Free Farming
Starting in 2025, Rodale Institute has begun investigating alternative forms of organic fertility, including livestock manure, leguminous green manures, and food waste processed through anaerobic digestion with the goal of creating closed-loop, energy independent, and carbon neutral or negative farming systems. At the global scale, by-products from food systems, particularly manure and food waste contribute 2 percent and 6 percent of all GHG emissions, respectively [25]. Emissions from crop residues account for an additional 1 percent of all emissions [26]. Anerobic digestion (AD), a natural process by which microorganisms decompose organic material under low oxygen conditions and produce biogas, has emerged as a win-win solution with the capacity to reduce GHG emissions from by-products of agriculture and food systems while simultaneously creating a source of renewable energy. Biogas from AD can be used locally for combined heating and power or as a fuel to power agricultural equipment. The digestion process creates a slurry fraction referred to as digestate, which can be used as organic fertilizer[27].
While AD has widely been shown as a viable emissions reduction strategy for food waste and crop residues in general, there has been little attention given to use of AD for crop residues from green manures specifically. Green manures are typically used in organic systems to provide fertility to the following cash crop. Early research has shown that AD of green manures may have synergistic effects on crop production and greenhouse gas emissions [28, 29] through biogas generation and the creation of an N-rich digestate as fertilizer. The potential of AD from green manures may be especially beneficial for low-input organic cropping systems, which typically rely on green manures exclusively for fertility, or farms that lack access to livestock manure. Currently, the high cost of AD installation often prevents small- and medium-size farms from investing in this technology, but small, containerized systems now exist that can be tailored to different sized farming operations. While larger scale projects at the farm or community level have the potential to not only generate greenhouse gas offsets but convert methane to a form of natural gas that can power agricultural equipment. Currently, there are several models of tractors that run on natural gas and could be utilized for large-scale grain operation. Through the integration of anaerobic digestion of agricultural waste products and green manures that can be produced on-farms, and the resulting bio-digestate as an organic fertilizer, the potential exists to eliminate the need for fossil fuels in agriculture and create fossil fuel free, carbon neutral cropping systems.
Conclusion
Large-scale conversion of farmland from conventional to organic production may be a viable solution to reduce current ambient CO2 levels in the short-term through soil carbon sequestration and lower greenhouse gas emissions. However, there is a need to increase organic crop yields in a manner that is not wholly reliant on livestock manure or other sources of fertility that will increase greenhouse gas emissions. Anaerobic digestion of agricultural waste products and green manures could be a viable solution to offset greenhouse gas emissions from the manufacture and application of fertilizers while at the same time increasing crop yields. There is potential that as technology emerges that this could result in fossil fuel free, carbon neutral or negative yet highly productive farming systems.
References:
1. Reganold, J.P. and J.M. Wachter, Organic agriculture in the twenty-first century. Nature plants, 2016. 2(2): p. 1-8.
2. Gattinger, A., et al., Enhanced top soil carbon stocks under organic farming. Proceedings of the National Academy of Sciences, 2012. 109(44): p. 18226-18231.
3. Squalli, J. and G. Adamkiewicz, Organic farming and greenhouse gas emissions: A longitudinal US state-level study. Journal of Cleaner Production, 2018. 192: p. 30-42.
4. Drinkwater, L.E., P. Wagoner, and M. Sarrantonio, Legume-based cropping systems have reduced carbon and nitrogen losses. Nature, 1998. 396(6708): p. 262-265.
5. Hepperly, P., et al., Organic farming enhances soil carbon and its benefits. 2007: CRC Press: Boca Raton, FL, USA.
6. Hepperly, P., et al., Compost, manure and synthetic fertilizer influences crop yields, soil properties, nitrate leaching and crop nutrient content. Compost Science & Utilization, 2009. 17(2): p. 117-126.
7. Littrell, J., et al., Long‐term organic management combined with conservation tillage enhanced soil organic carbon accumulation and aggregation. Soil Science Society of America Journal, 2021. 85(5): p. 1741-1754.
8. Lorenz, K., et al., Soil organic carbon and total nitrogen after 34 years under conventional and organic management practices at the Rodale Institute Farming Systems Trial. Soil Science Society of America Journal, 2025. 89(1): p. e14165.
9. Venkat, K., Comparison of twelve organic and conventional farming systems: a life cycle greenhouse gas emissions perspective. Journal of Sustainable Agriculture, 2012. 36(6): p. 620-649.
10. Skinner, C., et al., Greenhouse gas fluxes from agricultural soils under organic and non-organic management—A global meta-analysis. Science of the total environment, 2014. 468: p. 553-563.
11. Skinner, C., et al., The impact of long-term organic farming on soil-derived greenhouse gas emissions. Scientific reports, 2019. 9(1): p. 1-10.
12. Leifeld, J. and J. Fuhrer, Organic farming and soil carbon sequestration: what do we really know about the benefits? Ambio, 2010. 39(8): p. 585-599.
13. Willett, W., et al., Food in the Anthropocene: the EAT–Lancet Commission on healthy diets from sustainable food systems. The Lancet, 2019.
14. Seufert, V. and N. Ramankutty, Many shades of gray—The context-dependent performance of organic agriculture.Science advances, 2017. 3(3): p. e1602638.
15. Hoffman, E., et al., Energy use and greenhouse gas emissions in organic and conventional grain crop production: Accounting for nutrient inflows. Agricultural Systems, 2018. 162: p. 89-96.
16. Chiriacò, M.V., S. Castaldi, and R. Valentini, Determining organic versus conventional food emissions to foster the transition to sustainable food systems and diets: Insights from a systematic review. Journal of Cleaner Production, 2022. 380: p. 134937.
17. Pearsons, K., Chase, C., Omondi, E., Zinati, G., Smith, A., Rui, Y. , Does reducing tillage improve the profitability of organic and conventional agricultural systems? Results from a long-term field trial. Sustainability (under review), 2022.
18. Barbieri, P., et al., Biological nitrogen fixation of legumes crops under organic farming as driven by cropping management: A review. Agricultural Systems, 2023. 205: p. 103579.
19. Pearsons, K.A., et al., A tale of two systems: Does reducing tillage affect soil health differently in long-term, side-by-side conventional and organic agricultural systems? Soil and Tillage Research, 2023. 226: p. 105562.
20. Wander, M.M., et al., Organic and conventional management effects on biologically active soil organic matter pools. Soil Science Society of America Journal, 1994. 58(4): p. 1130-1139.
21. Pimentel, D., et al., Environmental, Energetic, and Economic Comparisons of Organic and Conventional Farming Systems. BioScience, 2005. 55(7): p. 573-582.
22. Hanson, J.C., E. Lichtenberg, and S.E. Peters, Organic versus conventional grain production in the mid-Atlantic: An economic and farming system overview. American Journal of Alternative Agriculture, 2009. 12(1): p. 2-9.
23. Lotter, D.W., R. Seidel, and W. Liebhardt, The performance of organic and conventional cropping systems in an extreme climate year. American Journal of Alternative Agriculture, 2009. 18(3): p. 146-154.
24. Xia, Y. and M. Wander, Management zone‐based estimation of positive and negative nitrous oxide flux in organic corn fields. Soil Science Society of America Journal, 2022.
25. Crippa, M., et al., Food systems are responsible for a third of global anthropogenic GHG emissions. Nature food, 2021. 2(3): p. 198-209.
26. Abalos, D., et al., A review and meta-analysis of mitigation measures for nitrous oxide emissions from crop residues. Science of The Total Environment, 2022. 828: p. 154388.
27. Möller, K. and T. Müller, Effects of anaerobic digestion on digestate nutrient availability and crop growth: A review. Engineering in life sciences, 2012. 12(3): p. 242-257.
28. Tidåker, P., et al., Rotational grass/clover for biogas integrated with grain production–A life cycle perspective.Agricultural Systems, 2014. 129: p. 133-141.
29. Brozyna, M.A., et al., Effects of grass-clover management and cover crops on nitrogen cycling and nitrous oxide emissions in a stockless organic crop rotation. Agriculture, ecosystems & environment, 2013. 181: p. 115-126.
Additional References:
- Moyer, J., Smith, A., Rui, Y., Hayden, J. 2020. Regenerative Agriculture and the Soil Carbon Solution [white paper]. Rodale Institute.
- Lal, R. Managing soils for negative feedback to climate change and positive impact on food and nutritional security. Soil Science and Plant Nutrition 2020, 66, 1–9, doi:10.1080/00380768.2020.1718548.
- Berry, W. The Gift of Good Land: Further Essays Cultural and Agricultural; Counterpoint Press, 1981; ISBN 978-1-64009-169-6.
- The Search for Systems that Regenerate Agricultural Potential. In Research issues related to strategic planning for United States agriculture in a global setting: proceedings and minutes, thirty-sixth annual meeting of Agricultural Research Institute, October 7-9, 1987, Washington, D.C; Agriculture Research Institute (U.S.), Rodale, R., Eds.; Agricultural Research Institute: Bethesda, Md, 1987.
- Lal, R. Soil carbon sequestration to mitigate climate change. Geoderma 2004, 123, 1–22, doi:10.1016/j.geoderma.2004.01.032.
- Khan, S.A.; Mulvaney, R.L.; Ellsworth, T.R.; Boast, C.W. The Myth of Nitrogen Fertilization for Soil Carbon Sequestration. Journal of Environment Quality 2007, 36, 1821, doi:10.2134/jeq2007.0099.
- Lal, R.; Follett, R.F.; Stewart, B.A.; Kimble, J.M. SOIL CARBON SEQUESTRATION TO MITIGATE CLIMATE CHANGE AND ADVANCE FOOD SECURITY. Soil Science December 2007 2007, 172, 943–956, doi:10.1097/ss.0b013e31815cc498.
- Oldfield, E.E.; Bradford, M.A.; Wood, S.A. Global meta-analysis of the relationship between soil organic matter and crop yields SOIL 2019, 5, 15–32, doi:https://doi.org/10.5194/soil-5-15-2019.
- Global Carbon Project. Carbon Budget and Trends 2019.
- Schmidt, M.W.I.; Torn, M.S.; Abiven, S.; Dittmar, T.; Guggenberger, G.; Janssens, I.A.; Kleber, M.; Kogel-Knabner, I.; Lehmann, J.; Manning, D.A.C.; et al. Persistence of soil organic matter as an ecosystem property. Nature 2011, 478, 49–56.
- IPCC Climate Change and Land: an IPCC special report on climate change, desertification, land degradation, sustainable landmanagement, food security, and greenhouse gas fluxes in terrestrial ecosystems.; Intergovernmental Panel on Climate Change, 2020;